Presentation
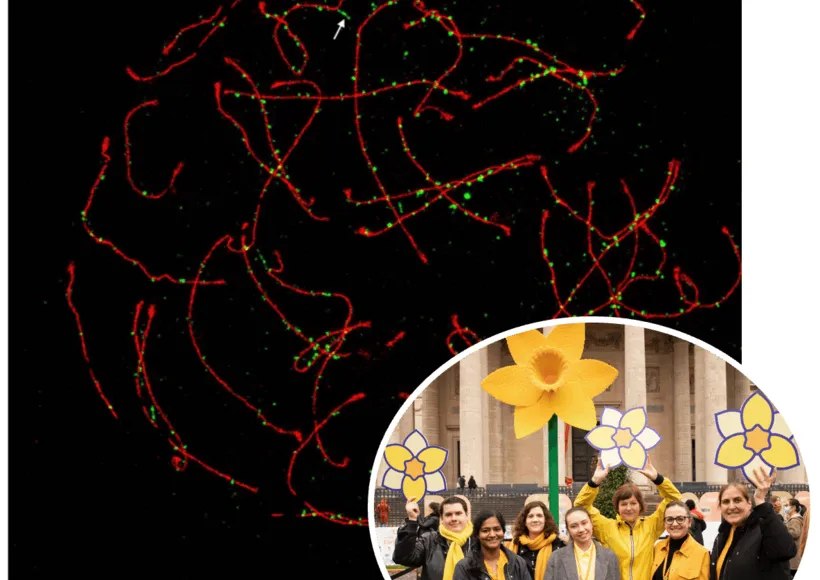
We study the process of recombination, that ensures genome stability and faithful chromosome segregation, during the production of haploid gametes at meiosis. Homologous recombination takes place when a broken or damaged chromosome uses a homologous sequence as a template for repair. Defects in homologous recombination have severe consequences and are linked to several disorders, such as certain inherited cancers and genetic instability syndromes.
Recombination can be due to accidental lesions, following exposure to genotoxic agents or replication failures, but lesions can also be deliberately self-inflicted by the cell, such as during meiosis. During meiosis, the genome is halved, with only one of each pair of parental homologous chromosomes being transmitted to progeny for sexual reproduction. Recombination ensures that physical links between homologous chromosomes, called crossing over, are created, necessary for their correct segregation during the first meiotic division. Errors in the meiotic recombination process lead to sterility or aneuploidy and are a major cause of chromosome abnormalities, such as in Downs Syndrome.
Recombination is initiated by programmed double-strand break (DSB) formation (Fig. 1A) along chromosomes, at hotspots whose determinants are poorly understood. Furthermore, only a subset of DSBs is processed to undergo crossing over, involving an important decision at the time of DSB repair. We study the factors that control DSB and crossing over formation and, in particular, the influence of chromatin, chromosome and nuclear organization on their positioning. One remarkable feature of meiosis is its conservation between species. Our model organism is the budding yeast Saccharomyces cerevisiae, among the closest to mammals in terms of meiotic recombination mechanisms and which allows a large panel of molecular, genetic and biochemical approaches.
Meiotic chromosomes are organized into linear arrays of loops anchored at their base to the chromosome axis structure. We have recently found that a chromatin modification, histone H3K4 methylation, promoted by the Set1 complex, is important for DSB formation. We have further shown that a sub-unit of the Set1 complex directly binds H3K4me3 through a PHD finger module and interacts with the proteins necessary for DSB formation on the axes, making the molecular bridge between these two chromosomal regions for DSB formation (Figure 1B).
We also investigate which mechanisms drive the repair of meiotic DSBs through crossover versus noncrossover. By genome-wide mapping of a crossover-associated protein, Zip3, we have uncovered specific chromosome features associated with high or low crossover frequency. We are currently applying proteomic approaches to decipher protein complexes involved in crossover repair of meiotic DSBs.
Finally, we study how chromatin is modified upon DSB formation. We found that two histone chaperones CAF-1 and Hir, are specifically recruited to meiotic DSBs, implying that new histones are incorporated into chromatin to replace those evicted by recombination, potentially altering the local composition of chromatin.
Our research should allow better understanding of the mechanisms allowing faithful transmission of genetic information during reproduction and also shed light on the involvement of chromatin and its modifications in somatic DSB repair by recombination, potentially contributing to epigenetic changes underlying disease and abnormal development.