Presentation
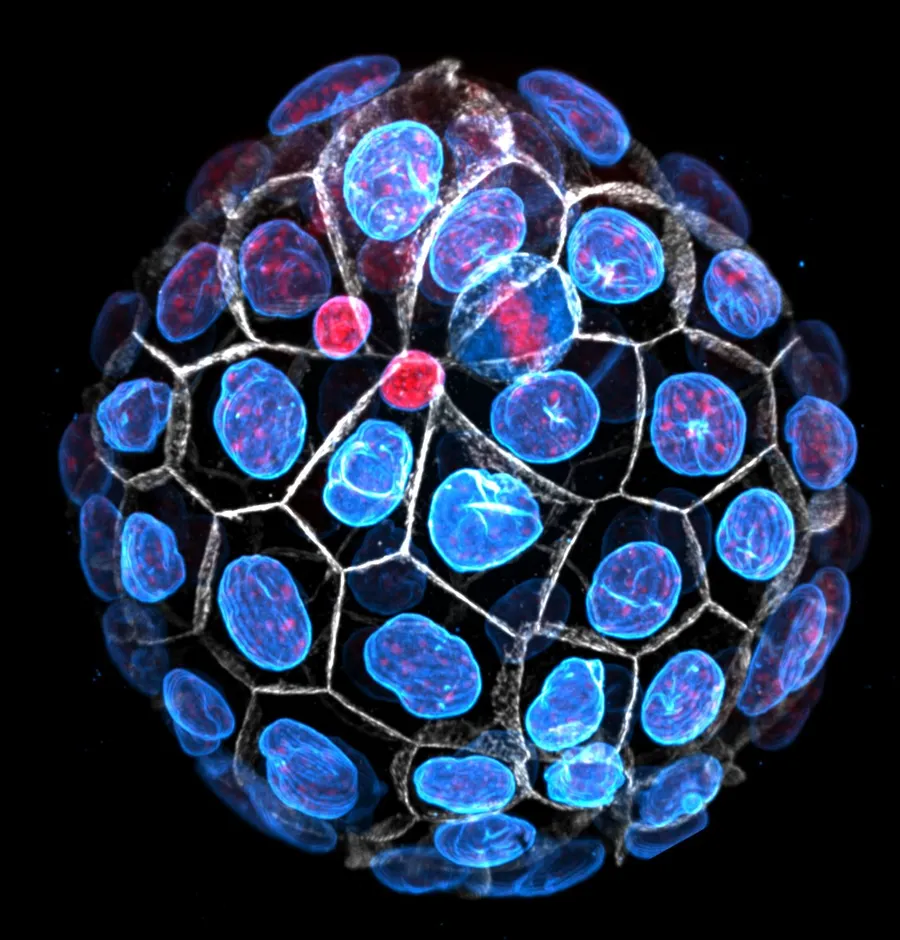
We want to understand how the mammalian embryo shapes itself. For this, we study how the forces that deform the embryo are generated. Usual suspects are the cytoskeleton, adhesion molecules and intercellular fluid. We use microscopy to observe the deformations, biophysical tools to measure the forces, and genetics to perturb them.
99SEC to understand developmental biology (video in french)
Podcast France Inter In Utero Les sept premiers jours et La danse des cellules (in french)
Award from the Fondation Bettencourt Schueller (video in french)
Seminar at Cité des sciences et de l'industrie (video in french)
Award from the CNRS (video in french)
ERC Starting Grant (video in english)
Talk at College de France (video in english)
Talk at College de France (video in english)
Talk Multiscale integration in biological systems (video in english)
During embryonic development, cells use the information contained in the genome to build the organism. This is accomplished through a succession of morphogenetic movements during which cells divide, die, deform and move. It is the sequence and combination of morphogenetic movements that build the specific architecture of each species. To understand morphogenesis, we need to consider evolution, genetics, cell biology and biophysics. Our research focuses on the mechanical aspects of mammalian embryo morphogenesis.
Morphogenesis of the preimplantation embryo
Preimplantation development gives rise to the blastocyst, which is composed of a squamous epithelium enveloping a cavity and the inner cell mass. To form the blastocyst, a sequence of three morphogenetic movements occurs: compaction, internalisation and cavitation.
Pre-implantation development
Timelapse of pre-implantation development of the mouse embryo (snapshots taken every 15 min)
Compaction
Timelapse of compaction of the mouse embryo (snapshots taken every 15 min)
At the 8-cell stage, compaction is a developmentally-controlled cell-cell adhesion process that rounds up the embryo. Similarly to the rounding up of a liquid droplet, compaction is driven by the surface tension of the cells. The surface tension of the embryo increases during the 8-cell stage as the actomyosin cortex strengthens. At the same time, this increase in contractility initiates periodic contractions that travel around the cell surface. See Pulsatile cell-autonomous contractility drives compaction in the mouse embryo.
Internalization
Timelapse of the internalisation of a chimeric doublet of cells expressing an actin marker (LifeAct) in different colours (snapshots taken every 15 min)
At the 16-cell stage, cells can adopt either an inner or outer position within the embryo. The 8- to 16-cell stage division is instrumental in determining the position of individual cells. This division can be asymmetric, as daughter cells acquire distinct components giving them different contractile properties. See Asymmetric division of contractile domains couples cell positioning and fate specification. These different contractile properties result in the self-organised sorting of the cells in either inner or outer position. See Adhesion functions in cell sorting by mechanically coupling the cortices of adhering cells. The positioning of the cells controls their genetic program and their differentiation into embryonic or extra-embryonic tissues. In addition, cell mechanics seem to influence this differentiation. See Asymmetric division of contractile domains couples cell positioning and fate specification.
Lumen formation
Timelapse microscopy of LifeAct-GFP (green) mTmG (magenta) embryo turning 32-cell stage and forming the blastocoel (snapshot taken every 10 min).
At the 32-cell stage, a fluid-filled lumen forms between the outer and inner cells and sets the first axis of symmetry of the embryo. During this process, myriad lumens grow between cells and the fluid eventually collects into a single lumen. The fracturing of cell-cell contacts results from pressurized fluid rushing from the extraembryonic medium into the intercellular space. During this process, cell-cell adhesion molecules provide mechanical resistance and can direct the final position of the lumen. In addition, the differences in the mechanical properties of the outer and inner cells squeezes the fluid to the periphery of the embryo rather than at its center. See Hydraulic fracturing and active coarsening position the lumen of the mouse blastocyst.
Periodic cortical waves of contraction
During morphogenesis, actomyosin contractility is a powerful engine of cell and tissue shape changes. Although these shape changes appear continuous, they actually are powered by pulses of contractions, which repeat periodically. Interestingly, these cyclic contractile events are conserved in evolution and can take various forms. In the mouse embryo, periodic contractions begin at the 8-cell stage, at the onset of compaction. See Pulsatile cell-autonomous contractility drives compaction in the mouse embryo.
Periodic contractions of the 8-cell stage mouse embryo
Timelapse of periodic contractions of an 8-cell stage mouse embryo in the absence of calcium (snapshot taken every 5 s).
Periodic cortical waves after asymetric division
Timelapse of periodic cortical waves of contraction (snapshot taken every 5 s). 16-cell stage doublet resulting from the asymmetric division of an isolated 8-cell stage blastomere. Membrane is shown in magenta and LifeAct-GFP in green. The unpolarised blastomere on top shows prominent periodic cortical of contraction while the polarised sister cell on the bottom remains quiet. The polarised blastomere will later envelop its unpolarised sister cell, which will become embryonic tissue.
Measuring cell and tissue mechanics of mammalian embryos
A micropipette aspiration set up can easily be coupled to high-resolution fluorescence confocal microscopes to relate cell mechanics to sub-cellular processes. See Adhesion functions in cell sorting by mechanically coupling the cortices of adhering cells.
Micropipette aspiration can be used to measure the surface tension, pressure and viscosity of cells and tissues. See Pulsatile cell-autonomous contractility drives compaction in the mouse embryo.
Using a dual pipette aspiration assay, adhesion strength can be measured in vitro. See Adhesion functions in cell sorting by mechanically coupling the cortices of adhering cells.
Separation force measurement
8-cell stage blastomeres are separated by pulling the micropipettes apart while applying increasing pressures in a stepwise fashion with the right-hand side micropipette.