Presentation
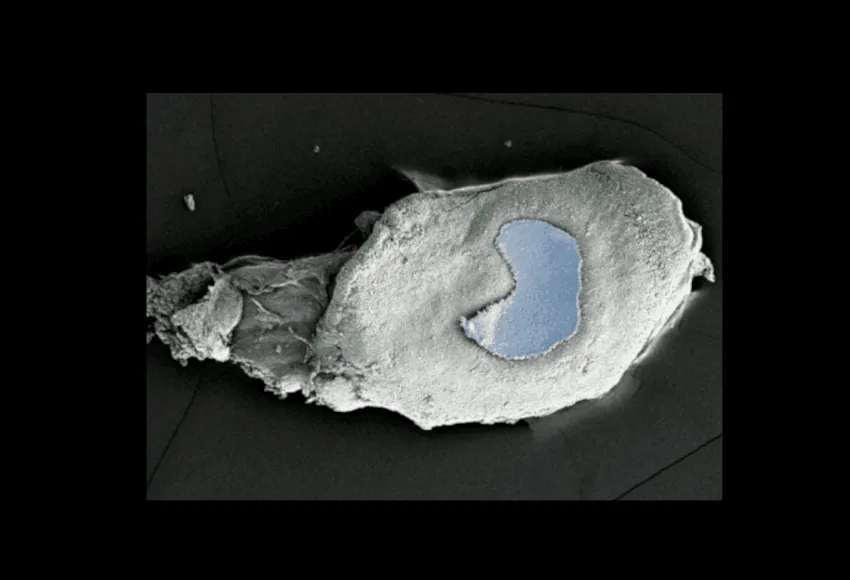
Team Martin (2023)
Hearing is initiated in the inner ear by mechanical vibrations of mechanosensory “hair cells”.
Hair cells respond to sound-evoked mechanical vibrations by producing electrical signals that then run along the auditory nerve to the brain. Our team develops experiments at the interface between physics and biology to shed light, at the scale of the single hair cell and of its molecular constituents, on the mechano-electrical transduction process that shapes our perception of sound at the periphery of the auditory system.
1. Active mechanics and motility of the hair bundle.
Auditory detection starts with the deflection of the hair bundle, the mechanical antenna of the hair cell that projects from the cell’s apical surface into the surrounding fluid (Fig. 1). The hair bundle comprises a few tens to a few hundres cylindrical villosities called stereocilia. Mechano-sensitivity of the hair bundle stems from direct mechanical activation of ion channels by tension changes in oblique tip links that interconnect neighbouring stereocilia within the bundle.
Our experiments demonstrate that the hair bundle behaves not only as a mechanosensory antenna but also as a sort of oscillatory micro-muscle that can actively amplify the hair cell’s responsiveness to sinusoidal stimuli (Martin et Hudspeth, 1999). Active hair-bundle motility can result in spontaneous oscillations (see video 1 in “Our videos”), which provide a cellular substrate for oto-acoustic emissions—the unprovoked emanation of sound from the ear. Elastic coupling between hair cells enhances the sensitivity and the frequency selectivity of auditory detection so that the sensory unit of the inner ear for the detection of a particular sound frequency is composed of a few tens of hair cells (Barral et al, 2010). The hair-bundle amplifier offers double benefit for hearing: it enlarges the range of sound intensities that can be heard by amplifying only the weakest sounds and sharpens frequency selectivity by filtering the input to the hair cell. Interestingly, the ear does not work as a high-fidelity sound receiver, introducing “phantom tones” that are not present in the acoustic input. We showed at the single-cell level that the hair-bundle amplifier produces distortions with physical properties that are characteristic of the phantom tones that are perceived in human hearing (Barral and Martin, 2012).
To interpret quantitatively our observations, we have built a theoretical description of active hair-bundle motility that is based on a dynamic interplay between mechanosensitive ion channels, molecular motors that actively pull on the tip links, and feedback by the calcium influx into the hair cell through the transduction channels (Nadrowski etal, 2004;Tinevez et al., 2007; Bormuth et al., 2014). Gating of the transduction channels produces internal forces that effectively reduce hair-bundle stiffness and increase hair-bundle friction (Bormuth et al., 2014; Barral et al., 2018; see video 2 in “Our videos”). Gating forces can be so large as to result in negative bundle stiffness, a mechanical instability that foster motor-driven oscillation of the hair-cell bundle and in turn mechanical amplification (Fig. 2).
Our results over the past 20 years promote a general principle of sound detection that is based on nonlinear amplification by self-sustained “critical” oscillators in the inner ear (Hudspeth et al, 2010): within this framework, each hair cell is thought to operate near an oscillatory instability—a Hopf bifurcation, which providesa characteristic frequency near which its response to sound is greatly amplified.
An active oscillator is ideally suited for hearing, but only for detection near its characteristic frequency of oscillation. Processing complex sounds such as those relevant to speech or music calls for the operation of a set of oscillators with characteristic frequencies that span the auditory range. Indeed, hair cells are spatially distributed in hearing organs according to a frequency (or tonotopic) axis, so that different sound frequencies are detected by different cells. Determining the mechanism that tunes the characteristic frequency of the hair cell over the auditory range (20 Hz to 20 kHz for human hearing) remains a major challenge for ongoing and future experiments. In this respect, we have recently demonstrated stiffness and tension gradients of the hair bundle as well as of the tip link’s molecular complex along the tonotopic axis of the mammalian cochlea (Tobin et al., 2019). Mechanical tuning of the inner constituents of the cochlea appears to happen at many scales: from the mesoscopic scale of the basilar and tectorial membranes that couples tens of hair cells together, to the cellular scale of the hair bundle and hair-cell soma, down to the molecular scale of the hair cell’s transduction apparatus.
In the long term, this fundamental research may serve as a guiding framework to design novel devices for patients suffering from severe sensorineural hearing loss.
2. Mechanical activity of the acto-myosin system—biomimetic oscillations.
In a complementary bio-mimetic approach to the hair-bundle oscillator, we have also shown in vitro with a minimal set of purified proteins that autonomous mechanical oscillations can emerge from the collective properties of molecular motors (Fig. 3A; Plaçais et al, 2009). We are currently developing experiments to identify and control the biophysical parameters that determine the active oscillatory behaviour of acto-myosin systems. In addition, we have also recently studied active transport and positioning of small cargos by small groups of molecular motors in controled networks of cytoskeletal filaments (Fig.3B; Richard et al., 2019).