Team
Replication program and genome instability
Thematic areas of research:
Image
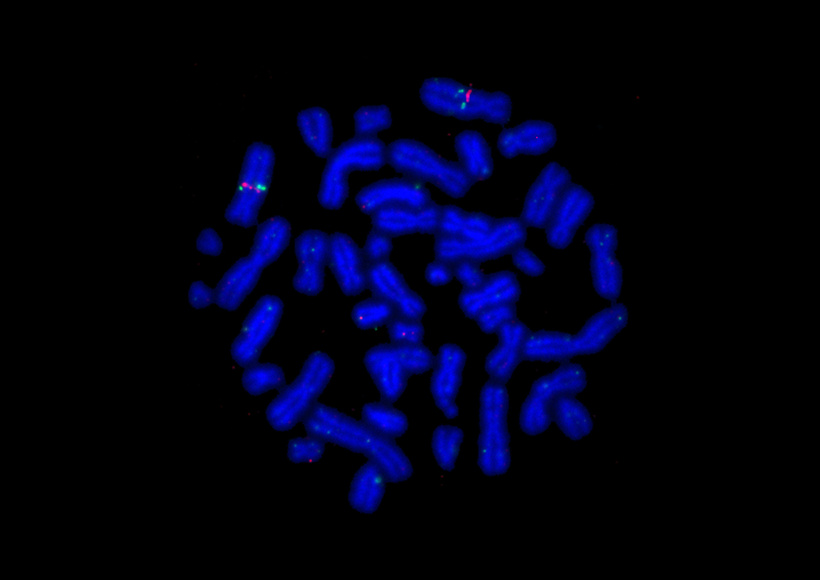
To top
Team
CHUNLONG CHEN
Replication program and genome instability
DNA replication must adapt to changes in chromatin organization associated with cell differentiation and development, whose deregulation can challenge genome stability and leads to mutations, cancer and many other genetic diseases. However, despite intensive studies, the mechanisms that coordinate where and when replication initiates in the human genome remain poorly known. Our team focuses on using cutting-edge high-throughput genomic approaches and genome-wide data analyses to study the spatio-temporal replication program of the human genome and its impact on genome stability, in particular to address the following questions:
- What determines the replication program, i.e. the position, the time of firing and the efficiency of replication origins in the human genome?
- How this program is regulated and associated with gene transcription and chromatin organization?
- How deregulation of these programs challenges genome stability and leads to human diseases?
Members
Image

Past members
Stefano GNAN
Post-Doctoral Researcher
Xia WU
Post-Doctoral Researcher
Sami EL HILALI
Bioinformatic Scientist
Yaqun LIU
PhD Student
Christelle LALANNE
Research trainee
Key publications
All publications
-
-
OKseqHMM: a genome-wide replication fork directionality analysis toolkitNucleic Acids Research
-
-
-
Kronos scRT: a uniform framework for single-cell replication timing analysisNature Communications
-
-
-
The impact of transcription-mediated replication stress on genome instability and human diseaseGenome Instability & Disease
-
-
Replication landscape of the human genomeNature Communications
News
All news
-
Unprecedented: a team from Institut Curie discovers how DNA unzips at the time of replicationAn article published in Cell and co-authored by Chunlong Chen, head of the Replication program and genome instability team at Institut Curie, reports an unprecedented discovery on DNA replication. It helps us better understand how life is created and gives hope for progress in oncology.
Since the discovery of the structure of the double DNA helix in 1953, the way in which double09/01/2023 -
Chunlong Chen, first Institut Curie’s recipient of the Fondation Bettencourt Schueller Impulscience program.The Fondation Bettencourt Schueller has chosen the research project of Chunlong Chen, group leader at Institut Curie Research Center, as one of seven recipients of its new research support program, Impulscience. This funding should allow him to extend his work in fundamental biology on the regulation of DNA replication, as well as genome transcription.28/11/2022
-
A next-generation method for examining DNA replication using “single molecule” analysisSuccessful replication is essential to maintaining genome integrity. At Institut Curie, a totally new “optical replication mapping” method has just been perfected by Chunlong Chen’s “Genome Replication and Instability Program” team as part of an international collaboration.13/07/2021
-
Genomic instability and pre-cancerous lesions: the role of R-loops gradually revealedIn the cellular construction process, DNA transcription and replication mechanisms can sometimes collide. These collisions lead to errors in DNA replication in the cell, and resulting defective cells can then lead to cancer.04/09/2020
-
Une origine importante de fragilité de l’ADN enfin élucidéeLes Common fragile sites (CFSs), ou sites fragiles communs en français, sont des fragments d’ADN qui peuvent facilement se casser lorsqu’elles sont soumises à de fortes contraintes, comme lors du « stress » que représente la réplication. Des chercheurs de l’Institut Curie ont étudié l’une des origines de cette instabilité.16/12/2019