Presentation
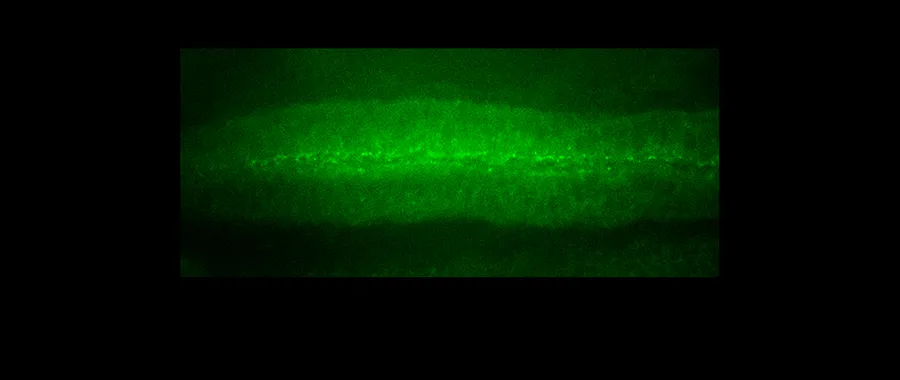
The Mechanics and Genetics of Embryonic and Tumour Development team studies the role of mechanical strain and deformation of macroscopic biological structures at the cell or multi-cellular scale, into the regulation and the generation of active biochemical processes at the microscopic molecular scale, including gene expression, in vivo. The group found the mechanical control of cell differentiation, and focuses on the coupling between mechanical strains and biochemical signalling in developmental and cancer biology.
The team also concentrates on the evolutionary emergence of the morphological and biochemical patterns at the origin of the first metazoans over 600 million years ago. The experimental results of the team indicate it as a as a mechanotransductive primitive behaviorial feeding response of pre-metazoa multi-cellular colonies to environmental marine flow hydrodynamic strains.
Our findings chronologically goes from the mechanical induction of cell trans-differentiation, by the modulation of the endocytosis of signalling proteins as a mechanotransductive underlying molecular mechanism (early 2000’s), to its role in the involvement of mechanical cues in the trigger of early Drosophila embryos mesoderm invagination (late 2000’s). It additionally goes from our finding of the mechanical induction of early Drosophila embryos endomesoderm differentiation through the mechanosenstivity of the beta-catenin pathway (from early to late 2000’s), which we most recently found as at the possible evolutionary origins of endomesoderm and first metazoa emergence (2010-20’s). A process anomalously reactivated as a tumorigenic signal in healthy epithelial tissues compressed by tumour growth pressure in vivo (2010’s).
Gastrulation and mesoderm formation are mechanotransductively triggered by soft internal fluctuations of cell shape
Gastrulation consists in the formation of large domains of tissue that internalize into the early embryo often like tubes, and which will develop as the internal organs of the adult animal, like the digestive tracks, or the heart, muscles and the kidney and the lung for most complex animals. In the Drosophila embryo, the first tube to form is the mesoderm, from which will derive all internal organs of the adult organism, except the digestive track. It forms thank's to the apical stabilisation of the molecular motor Myo-II at the external embryonic surface of the cell, which has the function of constricting the external surface of the embryonic tissue, thereby inducting the inward curvature of the tissue leading to the internalisation of the mesodermal tube. This constriction follows two phases. During the first phase, cells constrict in an erratic and unstable way in a second phase, due to the erratic and unstable formation of Myo-II spots at the mesoderm cells apexes. Then, cells constrict in a stable and coordinated way, due to the stabilisation of the Myo-II spots progressively reaching cell apexes.
We have demonstrated that the mechanical constraints developed by the stochastic fluctuations of shape of the apexes of the first phase activate the transition to the apical stabilisation of Myo-II second phase, thereby triggering the active process of mesoderm invagination (Mitrossilis et al, Nature Communications 2017).
To do so, we have used a mutant (of the snail gene) in which mesodermal cells do not fluctuate anymore, and which does not show any mesoderm invagination (mutant of snail). We have mimicked apex shape fluctuations with the amplitude of 500 nm only, by magnetic means. Indeed, we have injected magnetic liposomes inside mesodermal cells and have approached at a few microns a network of micro-magnets which individual size, of 10 microns, is on the order of magnitude of the individual cell size. The specificity of the local magnetic field produced by these magnets was to vary with time, controlled by the experimentalist, so that we made oscillate the local micrometric magnetic fields in such a way cells apex began to pulsate quantitatively like in the non mutated embryo (Figure 1A-left). In response to this stimulation, we have observed the stabilisation of Myo-II and the trigger of mesoderm invagination (Figure 1A-right). This stimulation is due to a mechanical activation of biochemical reactions, which we have identified as the activation of the Fog signalling pathway.
In addition, we have shown, by coupling genetic and magnetic tools again, that the mechanical deformation, this time induced by the mesoderm invagination on the cells of the endoderm of the posterior pole of the embryo (the future embryonic posterior gut track), triggers the apical stabilisation of Myo-II and initiate the posterior gut track formation. Which shows a mechanotransducive self-induced cascade initiated by snail-dependent pulsations at the origin of mesoderm and endodem gastrulation morphogenetic movements (Mitrossilis et al, Nature Communications 2017).
Furthermore, we find Twist, the gene product initiating mesoderm specification, as mechanically induced by the morphogenetic movement of gastrulation. Indeed, in non gastrulaing snail mutants, Twist expression is strongly reduced compared to the WT (Figure 1B Left). In snail mutants mechanically stimulated to rescue the invagination (see Fig.1A and Fig.6), the mechanosenstive gene product Twist (see Fig.5A) is rescued (Figure 1B - right) (Bouclet, Brunet et al, Nature Comm. 2013). Interestingly, Twist is expressed in the cells having invaginating only: in case the invagination rescued is smaller than the WT one (Fig. 1B right down), Twist rescue is observed in the small invaginating tissue only. Indicating Twist mechanosensitivity as a coordinator of biomechanical with biochemical morphogenesis in embryonic development: only cells having mechanically experienced invagination will in the end differentiate into a mesoderm, in such a way mesoderm will not developp outside of the embryos by accident in case of fluctuation in the number of cells having invaginating during gastrulation.
The mechanical constraints of gastrulation cause the opening of the major site Y654 of beta-catenin interaction with E-cadherin that initiates its phosphorylation by Src42A and the activation of the downstream transduction pathway, leading to the expression of beta-catenin target gene twist that specifies endomesoderm differentiation
Simulations predict that under the effect of a 6pN force, the two alpha helixes connected by the interaction of Y654-β-cat with D665-Ecad expand by 1nm, with the site Y654 having a 15% chance of opening (Fig.2a). Such dilation was confirmed quantitatively experimentally in FLIM in response to mesoderm invagination (not shown) or to associated mechanical stresses mimicked by magnetic means in embryos defective in gastrulation (Fig. 2b). The site Y654 is then indeed made more accessible, by about 20% under stress, to its specific antibody (Fig. 2c) – and this consistently even more in the absence of Src42A responsible for its phosphorylation under mechanical stress and its release into the cytosol for transcription. This favours its release of junctions (not shown) (Röper et al, e-LIFE 2018), and stimulates the maintenance of twist gene expression during mesoderm invagination (see section just beyond and the “Evo-Devo” and “Developmental Biology” sections below, Desprat et al, Dev. Cell. 2008, Brunet, Bouclet et al, Nature Communications 2013).
The mechanical strains developed by tumorous growing tissues on compressed healthy neighbouring cells mechanically induces the expression of tumorous genes via Y654 beta-catenin phosphorylation
We found β-catenin dependent mechanical induction of oncogenes expression leading to hyperproliferation and tumour initiation in wild type and pre-tumorous heterozygous (Apc mutated , Apc being a mutation found in 85% of human coilon cancers) mice colon healthy epithelia respectively, in response to tumour growth pressure in vivo (M-E Fernandez-Sanchez, S. Barbier et al, Nature 2015, – Figure 3).
To do so, we mimicked the 1kPa tumour growth pressure in vivo by magnetically loading the mesenchymal conjunctive tissue with ultra-magnetic liposomes, which we submitted to a permanent magnetic field gradient due to a millimetric magnet sub-cutaneoulsy localized in front of the colon. Such mechanical strain activated the phosphorylation of the Y654-β-catenin leading to the release of a junctional pool into the cytoplasm. It additionally led to the phosphorylation of Ser9-GSK3β allowing the nuclear translocation of the cytoplasmic beta-catenin into the nucleus and the expression of its tumorigenic target genes. The same responses are observed in the non-tumorous crypts compressed by neighbouring Notch-hyperproliferative crypts of a mice model of tumour progression.
In this tumour setting, we found that the kinase that phosphorylates Y654-βcat is Ret. We found that Ret is furthermore itself activated (phosphorylated at its Y1062 site) mechanically, and is both upstream of the phosphorylation of Y654-βcat and of the inactivation of GSK3-β by its phosphorylation on Ser9 (M-E Fernandez-Sanchez, S. Barbier et al, Nature 2015).
Spontaneous myogenic pulses in the colon maintain physiological Stem Cell levels via mechanical activation of the Ret/βcat pathway, a process pathologically amplified by the added permanent pressure of tumour growth in the presence of tumours.
Blocking spontaneous myogenic pulses of 1kPa amplitude with a cannabinoid (Win) shows a 2-fold decrease in Stem Cell number in vivo, which is restored by applying pulsed magnetic pressure of the same amplitude, using magnetic technologies similar to those developed to produce tumour growth pressure in vivo (Figure 3). This recovery is blocked by the use of Ret inhibitors (Nguyen Ho-Bouldoires, Sollier, Zamvirof et al Comm Biol 2022, Figure 3-ii-A).
In normal mice, magnetically adding permanent 1kPa tumour growth pressure increases the number of Stem Cells by a factor of 1.5, and by a factor of 2 in the Apc mutated context (associated to 85% of human colon cancers, see paragraph beyond). This increase is at the origin of the mechanical induction of hyperproliferation and tumorigenesis. It is blocked by the Ret inhibitor Vandetanib (Nguyen Ho-Bouldoires, Sollier, Zamvirof et al Comm Biol 2022, Figure 3-ii-B).

Figure 3-iii: Acoustic mechanical stimulation of Ret activation and of Stem Cells formation (Lgr5 expression).
More recently, the use of focused ultrasound waves (FUS) has enabled us to observe mechanical stimulation of Ret activation and stem cell formation as well (Figure 3-iii, Zamvirof, Nguyen et al, Nat. Biomed. Eng., 2024).
Evo-Devo: a mechano-transductive origin of mesoderm emergence in the common ancestor of bilaterian complex animals
We found that the mechanical activation of the beta-catenin pathway, anomalously activated in the process of tumour development, is an ancestral property, having been probably involved in the emergence of first differentiation patterns in ancient organism embryos, such as in the evolutionary emergence of the mesoderm in the last common ancestor of bilaterians. We effectively demonstrated the conservation of mechanical induction as involved in early mesoderm differentiation in both the zebrafish and Drosophila embryo, initiated by the mechanotransductive phosphorylation of the Y654 site of beta-catenin impairing its interaction with E-cadherins, leading to its release from the junctions to the cytoplasm and nuclei, and subsequently to the brackury and twist earliest mesoderm target genes expression, respectively (Figure 4).
The evolutionary origin of mesoderm emergence remains a major persisting opened question of todays Evo-Devo. Our results allow to suggest mechanostransductive Y654 phosphorylation in response to first embryonic morphogenetic movements at the origin of mesoderm emergence in the 570 millions years ago last common ancestor of bilaterians (Bouclet, Brunet et al, Nature Comm. 2013).
Evo-Devo: a more ancient mechanotransductive origin of endomesoderm specification and morphogenesis in first metazoan by environmental hydrodynamic marine mechanical strains
More recently, we found mechanotransductive induction of the endomesoderm gene fz10 expression via mechanical activation of Y654 phosphorylation by the morphogenetic movement of gastrulation in the cnidarian sea anemona Nematostella vectensis (N. vectensis) (Fig.4-iiA), which common ancestor with the Bilateria dating back 600-700 million years. It is thought that endomesoderm patterning, ancestral to endoderm and mesoderm differentiation, probably specified the primitive gut tissues of first Metazoa over 700 million years ago.
We also found that mechanical stresses developed by marine hydrodynamic flow trigger gastrulation in early N.vectensis embryos in a Myo-II-dependent process (Fig.4-iiB), reminiscent of the mechanical stimulation of mesoderm invagination found in bilateria Drosophila embryos (Fig.1). In addition, we found Myo-II-dependent hydrodynamic stimulation of gastrulation-like inversion in Choanoeca flexa (Fig.4-iiC), a sister group to Metazoa whose common ancestor dates back more than 700 million years.
We proposed that this response was evolutionarily selected, since Choanoeca flexa fully inverted on themselves trap their prey that are in suspension thanks to marine movements, and feed three times better than non-inverted ones without marine flows. And that this response has, in fact, become behavioral, with Choanoeca flexa then capturing their prey once made more accessible in suspension by marine flows, perceiving these marine flows as a signal of more favorable feeding conditions. Finally, we proposed that genetically induced internal mechanical constraints (such as snail-dependent fluctuations in the Drosophila embryo) replaced environmental constraints and thus autonomously triggered the initiation of embryogenesis: gastrulation (see above) (M.N. Nguyen, T. Merle et al., Front. Dev. Cell Biol. 2022, ).

Figure 4-iii : Conservation of Myo-II-dependent hydrodynamic stimulation of gastrulation-like inversion in the last common pre-metazoan multi-cellular organism ancestor dating back to at least 700 million years ago.
Our results thus suggest that the emergence of endomesoderm formation in early metazoans might have been stimulated in a behaviourial mechanotransductive manner by environmental hydrodynamic marine mechanical constraints, leading to its Myo-II-dependent gastrulation, and to its subsequent gastrulation-induced Y654-bcat-dependent specification, in early metazoans (Figure 4-iii, M.N. Nguyen, T. Merle et al., Front. Dev. Cell Biol. 2022, Nguyen and Farge, Nat. Com., 2024).
Developmental Biology: mechano-genetic and mechano-proteic reciprocal coupling in the regulation of gastrulating embryos development
Embryonic development is a coordination of multi-cellular biochemical patterning and morphogenetic movements. Last decades revealed the close control of Myosin-II dependent biomechanical morphogenesis by patterning gene expression, with constant progress in the understanding of the underlying molecular mechanisms. We recently revealed reversed control of the Twist developmental differentiation patterning gene expression (Figure 5) and of Myosin-II active relocalisation (Figure 6) by the mechanical strains developed by morphogenetic movements at Drosophila gastrulation, through mechanotransduction processes involving the Armadillo/beta-catenin and the down-stream of Fog signalling pathways (due mechanical inhibition of Fog endocytosis in this case, see next paragraph), respectively.
We used experimental tools (genetic and biophysical control of morphogenetic movements, Figure 5,6), and theoretical tools (simulations integrating the accumulated knowledge in the genetics of early embryonic development and morphogenesis) (Figure 7), to uncouple genetic inputs from mechanical inputs in the regulation of Twist meso-endoderm gene expression and Myosin-II active relocalisation. Specifically, we set-up an innovative magnetic tweezers tool to measure and apply physiological strains and forces in vivo, allowing to mimic morphogenetic movements from the inside of the tissue in living embryos (Figure 4). Farge, Curr. Biol., 2003; Desprat et al, Dev. Cell. 2008; Pouille et al Phys. Biol. 2008; Ahmadi, Pouille et al, Science Signalling, 2009).
Endocytosis: vesicle budding driving force; mechanical modulation of endocytosis as a mechanotransduction process triggering transdifferentiation
Historically, the first main thematic studied in the team was the motor role of biological membrane soft matter elasticity into the budding driving force of vesiculation initiating plasma membrane endocytosis (Rauch et al, Bioph. J, 2000), as well as the role of mechanical inhibition of morphogene endocytosis in mechanical induction of cell transdifferentiation (Figure 8, Rauch et al, Am. J. Cell Phys, 2002).